Equine Protozoal Myeloencephalitis
Exploring Neurologic Disease
Equine Protozoal Myeloencephalitis (EPM) is the most common neurologic disease seen in horses referred to Ohio State. In fact, more than 10% of equine cases presented to the Veterinary Medical Center at Ohio State have neurologic disease.
Horse owners in Ohio may spend as much as an estimated $4 million a year in direct costs for diagnosis and treatment of this disease. Since 1993, the American Live Stock Insurance Company of Geneva, Illinois has funded most of the EPM research.
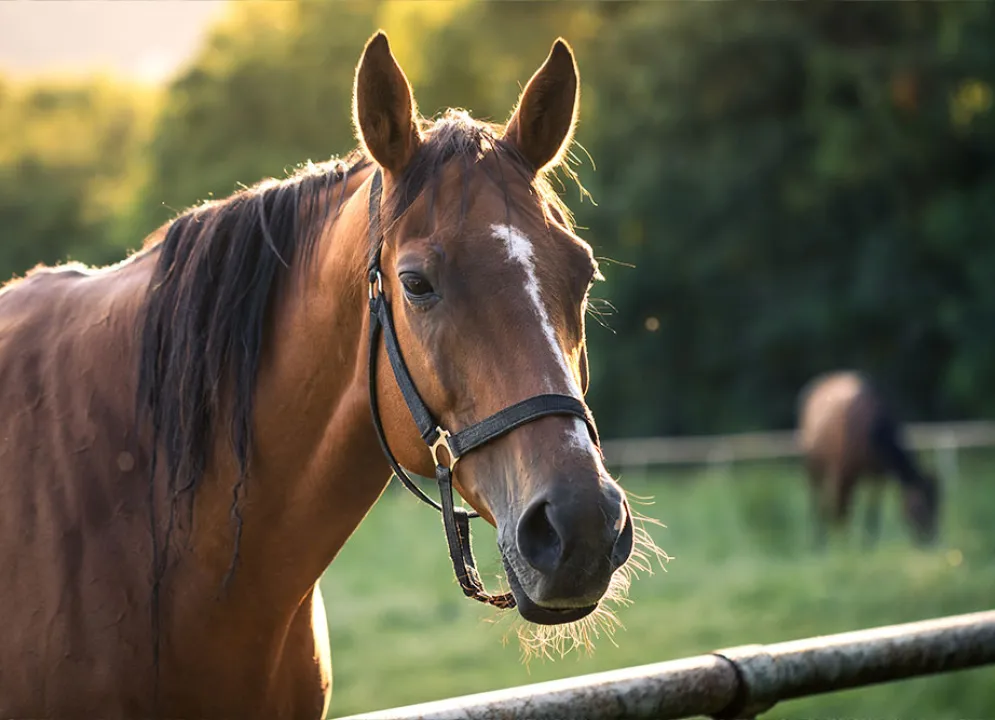
Equine Protozoal Myeloencephalitis Information
The clinical signs of Sarcocystis neurona can be quite variable. Variation of the clinical signs are a reflection of the focal, multifocal or diffuse nature of the lesions which occur randomly in the grey and white matter of the brain, brain stem or spinal cord. Usually the physical examination is within normal limits and the horses appear bright and alert, although focal muscle atrophy may be observed. The onset of clinical signs may be gradual, but more typically there are mild signs acutely with sometimes very rapid progression.
The neurological examination often reveals ataxia and incoordination in all four limbs which sometimes shows lateralization or there may be gait abnormalities with only one limb involved. Infection with Sarcocystis neurona can result in brain stem as well as spinal cord signs and often causes damage to the lower motor neuron of spinal cord or cranial nerves leading to muscle atrophy. The muscle atrophy is most common in the quadriceps and gluteal regions in the hind limbs, but also may mimic sweeney, radial paralysis or polyneuritis equi. If the brain stem is involved, atrophy of the temporal-masseter muscles and occasionally the tongue may be evident along with head tilt, facial nerve paralysis and difficulty swallowing. It is important to carefully examine for signs of muscle wasting as well as loss of sensation along the face, neck or body.
A frequent complaint is obscure lameness which can progress to ataxia, spasticity and incoordination of the limbs. Poor coordination and weakness are worsened by walking with the head elevated or walking up or down a slope. The asymmetric nature of the clinical signs is important to recognize as this can be an early indication of EPM. Horses with brain stem involvement often have head tilt, facial paralysis, loss of sensation of the cornea and internal nares as well as dysphagia, circling and acute recumbency. Some horses have a tendency to lean towards the wall of the stall to balance themselves. At least three horses have been observed with seizures as the only clinical signs. There are also reports of narcoleptic-like symptoms in some horses.
Differential diagnosis for horses suspected to have Sarcocystis neurona infection may be any disease affecting the central nervous system, although, depending upon the neuroanatomic localization certain problems may be more probable. That is to say, in a horse with weakness, ataxia and spasticity of all four limbs with no muscle atrophy or cranial nerve deficits, the horse may have cervical vertebral stenotic myelopathy or equine degenerative myeloencephalopathy. Both affect young horses (1-3 years of age), but CVM occurs more often in males. The clinical signs are often symmetric with the hindlimbs usually a grade worse than the forelimbs. The signs may be exaggerated by flexing or hyperextending the neck.
Equine herpesvirus myeloencephalitis (EHV1) often has an acute onset following an episode of fever, cough and nasal discharge or following one or more abortions on a farm. This condition often affects more than one horse on a farm. Herpesvirus has a rapid onset and often results in severe hindlimb weakness and ataxia along with bladder dysfunction. Urine dribbling may sometimes occur. The ataxia and weakness is usually symmetric and may result in recumbency. Occasionally the horses will dogsit. Cranial nerve involvement is not common.
Another disease which must be considered as a differential for EPM is Polyneuritis Equi. This disease can occur both acutely and insidiously. It is more common in mature horses and usually starts with hyperesthesia progressing to anesthesia. There is progressive paralysis of the tail, rectum, bladder and urethra leading to urine dribbling. Rear limb ataxia with gluteal atrophy may be present. Asymmetric cranial nerve deficits with involvement of cranial nerves five, seven and eight have been reported in 50% of the cases.
Verminous myeloencephalitis should also be considered as the signs are extremely variable depending on the parasite's migratory pathway. Diffuse or multifocal brain and spinal cord lesions have been reported. The onset is usually sudden with rapid deterioration and death. The incidence of this disease is very low, perhaps due to more intense parasite control.
A majority of the information known is gathered from fnaturally occurring infections. Regional epidemiological studies of EPM have yielded dissimilar results. Although regional differences probably occur, the most reliable general information for North America was provided by an EPM workshop in 1988. It was based on 364 histologically confirmed cases from 10 different North American centers. In descending order of frequency, Thoroughbreds, Standardbreds, and Quarter Horses, were most often affected, although many other breeds and ponies were represented. Usually only one animal in a herd or location is affected, although recently there have been 3 reports of farms with higher incidence of EPM from Ohio and Kentucky. The age of affected horses ranged from two months to nineteen years but over 60% were four years old or less. No geographic or seasonal predilection could be established.
Preliminary seroprevalance data have been collected using immunoblot testing to detect antibodies to S. neurona-specific proteins in equine serum samples. Initial testing in Kentucky and Ohio detected an average exposure rate above 20% among clinically normal horses. Recent investigations conducted at the University of Kentucky laboratory on serum samples collected from horses in Ohio, Kentucky and Pennsylvania have demonstrated an exposure rate above 40% among clinically normal horses. Findings from our study regarding Ohio horses indicated an increase in seroprevalence with age and a decrease in seroprevalence with an increase in number of freezing days. Exposure rates on individual farms ranged from approximately 0% to 100%. In 1978, Cornell University reported that 25% of all their equine neurologic disease accessions were due to EPM. The number of cases diagnosed at the University of Kentucky, Lexington, KY, has increased from about 8% of all neurologic accessions to 15% over the last few years.
A recent report from The Ohio State University Veterinary Medical Center revealed a 25% incidence of EPM in all horses presented for spinal ataxia in 1992, increasing to 52.9% in 1995 based on Western Blot analysis of serum and CSF. It is difficult to determine if the increase is actual or due to heightened awareness caused by the availability of immunoblot testing of serum and cerebrospinal fluid (CSF). Horses that actually reach the post mortem room represent a small portion of the total number of horses affected annually. Samples processed for immunoblot testing at the Gluck Equine Research Center suggest that several thousand cases occur in the US each year.
Sarcocystis neurona has been cultured from CNS lesions of eight horses from several different locations: New York, California (3), Panama, and Kentucky (3). Preliminary morphologic, immunologic, and DNA comparisons have detected only minor differences among isolates. This stage of Sarcocystis spp. is not known to be transmissible to other animals. Transplacental infection has not been reported but cannot be ruled out. Many normal foals have been produced by EPM suspect mares. Recently one of the mares was euthanized and a diagnosis of EPM was histologically confirmed. Her foal, taken at euthanasia, died of pneumonia at eight weeks of age without neurologic signs. No lesions were present in the CNS. Although the mare was immunoblot positive in serum and CSF, the foal was immunoblot negative. The earliest EPM case reported occurred in a two month old foal. If transplacental transmission does not occur, the minimum incubation period may be eight weeks. However, a recent case suggests the incubation period may be much shorter. Serum and CSF collected 4 days after onset of clinical signs were both negative for antibodies to S. neurona. Serum and CSF collected 31/2 weeks later were both positive. This indicates that the parasite was ingested and caused clinical signs in the 10-12 days required to produce a detectable antibody response.
Sarcocystis spp. belong to the phylum apicomplexa which includes several genera of coccidia that utilize an obligatory, predator-prey life cycle. The host range for an individual species of Sarcocystis is usually narrow. Sarcocystis spp. produce sporulated oocysts in the gut wall of the appropriate predator or definitive host (opossum). However, the oocyst wall is very fragile and usually ruptures before being passed in the feces. Infective sporocysts are introduced into the food and water supply of the prey animal or intermediate host by fecal contamination from the predator. Birds and insects may serve as transport hosts to further disseminate sporocysts. Original work performed both in the laboratory and out using Sarcocystis gigantea sporocysts suggested that there were environmental factors that may affect the life of the sporocyst. It appears that the organism does not survive well in extremes of hot or cold, freezing and thawing, nor extremes of ultraviolet radiation. Nevertheless, there was speculation that the organism could survive and remain viable up to one year. Recent studies using Sarcocystis cruzi sporocysts, a different species, corroborates what was found in the earlier work, however, there are indications that the sporocysts may remain viable for shorter periods of time. Although these are not S. neurona sporocysts, the effects of climate may also apply, and therefore may affect transmission of the disease to your horse.
Once ingested by the intermediate host (birds), sporocysts release sporozoites which penetrate the gut and enter arterial endothelial cells in various organs. Meronts develop rapidly and eventually rupture the host cell releasing merozoites into the bloodstream. This is usually followed by a second round of merogony in capillary endothelial cells throughout the body. Second generation merozoites are released into the blood stream and usually enter skeletal muscle cells where they develop into specialized meronts known as sarcocysts. Mature sarcocysts contain bradyzoites which are only able to complete the life cycle when ingested by the appropriate predator or scavenger.
Sarcocysts of S. neurona have not been found in affected horses, precluding transmission of the parasite to the definitive host. The horse is an aberrant, dead-end host. Sarcocystis neurona probably cycles normally between 2 or more wildlife species. The list of animals that may serve as the true intermediate host(s) is extremely long. Although many species have been suggested as the true definitive host, skunks, raccoons, or opossums were the most probable. Like EPM, these species are unique to the Western hemisphere. Immunoblot testing of serum from several skunks demonstrated the presence of antibodies to S. neurona-specific proteins. Raccoons and opossums tested negative. Skunks may be inadvertently exposed, similarly to horses, but the absence of antibodies in other wildlife species suggests that parasites are penetrating the gut and stimulating an immune response in the skunk only.
In 1995, results of PCR analysis provided strong evidence that the opossum is the definitive host of Sarcocystis neurona. This study revealed a 99.67% homology with the opossum sporocyst, Sarcocystis falcatula. These results have been corroborated by others. Experimental induction of EPM has just been completed by investigators at the University of Kentucky, which further corroborates that the opossum is the definitive host for this organism. The opossum is indigenous to North, Central and South America which coincides with the fact that cases of EPM have only been reported in horses that have lived in areas that the opossum inhabits.
Additional research by investigators from California indicates that there may be other organisms that cause EPM. This work suggested that Neospora sp. may be one of those causes. Neospora is a worldwide cause of abortion particularly in cattle. In contrast, all previous research indicates that EPM is a disease of the Americas which is related to the definitive host, the opossum, who happens to be indigenous to the Americas. Therefore, the likelihood of Neospora being a major cause of EPM is very small.
Unlike most Sarcocystis spp., S. neurona (falcatula) may aberrantly infect a large number of intermediate hosts such as it does in the horse. Reports in the literature indicate infection with this organism may be possible in dogs, sheep, cats, mink, raccoons, striped skunks, golden hawks, rhesus monkeys and chickens. The natural intermediate hosts are cowbirds and grackles, however earlier work suggests that the organism may form sarcocysts in the muscle of many species of birds from several different Orders. This wide host range is atypical for Sarcocystis spp., however, this behavior is similar to Toxoplasma gondii which may be phylogenetically similar. This would suggest the possibility of more than one species of S. falcatula or perhaps there are subspecies. Recent work generated by the University of Florida would suggest that S. neurona is distinct from S. falcatula and that the natural intermediate host for S. neurona is not the cowbird or grackle. It would appear that the opossum does harbor more than one species of Sarcocystis. More recent evidence would suggest that the Opossum harbors at least three species of Sarcocystis. The three species likely include S. neurona, S. falcatula and S. speeri.
This information was generated using nude mice and gamma-interferon knockout mice, therefore, further research is needed to determine the effect of all three species in the horse. More research is needed to determine the exact life cycle of this organism. The most recent evidence regarding the life cycle of S. neurona has taken the top spot in the news. Back in the fall of 2000, it was demonstrated that experimentally the domestic house cat can be used to complete the life cycle of this organism. In this study, the sporocysts were administered to both IFN-gamma KO mice and to ponies resulting in death in the mice and seroconversion and neurologic deficits in the ponies.
As far as the cat role in nature, that is yet to be determined. Subsequent to the cat discovery, it was demonstrated that the nine-banded armadillo is naturally infected with S. neurona. Serum samples from 19 wild-caught armadillos were all positive for anti-S. neurona antibody. Sarcocyst-infected muscles from the armadillo were fed to laboratory raised opossums resulting in shedding of sporocysts. The sporocysts were administered to a two-month old foal resulting in immunoconversion and development of neurologic deficits. The latest intermediate host determination involves the striped skunk. The naive skunks were administered S. neurona sporocysts from a naturally infected opossum and subsequently developed sarcocysts in the muscle. Sarcocyst-infected muscle was then fed to laboratory-raised opossums which shed sporocysts in their feces. The sporocysts were the administed to IFN-gamma KO mice and a two-month old foal with similar results to what has been demonstrated previously with the cat-derived sporocyst. Since early work done by Granstrom demonstrated S. neurona antibody in wild-caught skunks, likely they do play a role in nature.
In addition to the many intermediate hosts, there is speculation that other vectors may be involved. It does not seem feasible that there are enough opossums to disseminate this organism all over the US. The birds are widely distributed across the country and very well may serve as transport vectors. As well, there are other suspected transport vectors such as the cockroach and perhaps other insects.
It seems likely that sporozoites penetrate the horses' intestinal tract, enter vascular endothelial cells, and must pass through the vascular endothelium of the blood-brain barrier to reach the CNS. It is uncertain whether merozoites pass through the blood-brain barrier within leucocytes or cross directly through the cytoplasm of endothelial cells.
The clinical severity of experimental sarcocystosis, in appropriate intermediate hosts studied, is directly related to the number of sporocysts fed. It appears that the ability of any individual to resist infection is related to the size of the infective dose, immune competency, environmental stress, and the species of Sarcocystis. Some individuals may be inherently more susceptible to infection, which may have variable heritability. It seems premature to speculate about this possibility until more is learned about the pathogenesis of clinical infection.
Equine protozoal myeloencephalitis (EPM) was initially identified by J.R. Rooney in 1964. The first cases were recognized among Standardbreds returning to Kentucky from racetracks in the Northeastern United States. Subsequent cases have been reported among native horses in most of the US as well as in Canada, Mexico, Argentina, Panama, and Brazil. As more cases were examined, it became apparent that the brain was frequently involved, thus changing the name to focal myelitis-encephalitis. Ten years later, a "Toxoplasma-like" protozoan was recognized in histopathologic sections and the disease gradually became known as EPM.
Dubey was the first to suggest that EPM was caused by a Sarcocystis organism. In 1991 he was able to culture the organism from the spinal cord of an affected horse and subsequently the organism was named Sarcocystis neurona because it often develops within neurons. Further work done by Dr. Granstrom and his group resulted in an antemortem diagnostic test for this disease using Western blot analysis to determine the presence of antibody to S. neurona in CSF.
Research completed in 1995 shed considerably more light on the life cycle through work done at the University of Kentucky and at the University of Florida. It was discovered through the use of PCR that the organism was very closely related to Sarcocystis falcatula which has the Opossum as it's definitive host. The natural intermediate hosts are the grackle and cowbird, although numerous other intermediate hosts may be affected. Further evidence for this organism being the causative agent of EPM was confirmed by experimental induction of the disease which was recently completed by the researchers at the University of Kentucky.
Immunoblot analysis of serum and cerebrospinal fluid (CSF) provides antemortem information about exposure to S. neurona. The test utilizes cultured merozoites to detect antibodies directed against proteins that are unique to S. neurona. Antibodies produced to other organisms can be differentiated. Immunoblot testing of CSF has demonstrated 89% specificity and sensitivity among approximately 300 neurologic cases that received post mortem examination. Positive CSF indicates that parasites have penetrated the blood-brain barrier and stimulated a local immune response. If the integrity of the blood-brain barrier is compromised, circulating antibodies may leak across and produce a false positive test result. False negative results have been rare, but may occur. Some horses may simply fail to respond to the S. neurona-specific proteins identified. The possible causes of false negative responses are important to consider so that affected horses are not misdiagnosed.
Horses that initially tested positive have become negative after several weeks of treatment and are apparently recovered. Chronically affected horses may test negative and still be infected or the horse may still exhibit neurologic signs. We speculate that this may be due to permanent CNS damage and that parasites are either no longer present or, antibody production is below test sensitivity. The use of PCR testing aids in parasite detection when antibody level is low. Acute cases that test negative should be re-tested in two to three weeks. However, the incubation period appears to be sufficiently long to allow production of detectable amounts of IgG before the onset of clinical signs in most cases. As was discussed before, one exception with a very short incubation period has been observed.
Use of PCR as a diagnostic tool has been developed. Using a random primed polymorphic DNA assay (RAPD), molecular comparisons were made between S. neurona and eight related coccidia, namely two Sarcocystis sp., one Toxoplasma sp. and five Eimeria sp. The DNA fragments from the S. neurona isolates had sequence homology, whereas, there was no sequence homology between the 550 bp of S. neurona and the other species of coccidia tested. It was therefore suggested that this S. neurona DNA fragment could be utilized as a species-specific probe for this parasite which will enable differentiation of S. neurona from other equine coccidia as well as having potential to help solve the life cycle by examining Sarcocystis sp. from possible definitive hosts. Further study was performed to determine the phylogenetic relationship of S. neurona to other members of the family Sarcocytidae. This was done based on the sequence of the small ribosomal subunit gene of S. neurona which was amplified using polymerase chain reaction (PCR).
This study led to development of species-specific amplification primers which could be utilized for wildlife testing to elucidate the life cycle of S. neurona as well as utility in antemortem diagnostics of EPM. Other research examined the specificity and sensitivity of polymerase chain reaction (PCR) for detection of S. neurona (falcatula) DNA in the CSF. The test was both sensitive and specific, however, it appears to be related to the presence of parasite in the CSF. In this study, there was a 40% sensitivity which is not unexpected as in most cases of EPM, the parasite is in the tissues, not free in the CSF. Therefore, it appears that the PCR testing may be useful prior to development of an antibody response in the CSF or in chronic cases where the antibody level has waned below detectable levels.
Other testing modalities have been used to help determine the disease status of neurologic horses. Previously, the CSF cytology, total protein, and CSF enzmes such as creatine kinase (CK) and aspartate aminotransferase (AST) were used to help diagnose neurologic diseases. Research at OSU would suggest that this information is of limited value, but may help determine if the CSF sample has been contaminated during the collection process. Other tests that appear to have more value in determining the true disease status of neurologic horses are the CSF indices.
These are calculations based on serum and CSF concentrations of albumin and immunoglobulin G. The calculations are called albumin quotient (AQ) and the IgG Index. The AQ will help determine the permeability of the blood-brain barrier (BBB) and helps to determine if the CSF sample collected was contaminated with serum or blood. The IgG Index will determine if the antibodies in the CSF were produced in the central nervous system (intrathecal) or whether they are due to leakage from the serum as well. Although the bugs have not been worked out conclusively, these tests may have an important role in augmenting clinicians in the diagnosis of EPM.
Sarcocystis neurona probably causes little pathology in the appropriate intermediate host, however, CNS lesions in the horse are often extensive. Lesions may be microscopic to several centimeters wide. The brainstem and spinal cord are affected most often. Microscopically, lesions are characterized by focal to diffuse areas of nonsuppurative inflammation and necrosis. Grey and/or white matter are affected. Organisms have been found in neurons, leucocytes, and vascular endothelium although they tend to develop most often in neurons.
There are no known definitive prevention programs for this disease at the present time. Most of the recommendations for control have been directed against the wildlife involved in the life cycle of Sarcocystis falcatula. At the present time, keeping opossums and other critters out of your barn away from your feed and bedding as well as methods to keep birds out, are the only measures we can recommend at this time. There are methods to carry out the removal of wildlife which can be discussed with your local wildlife officers.
Another method of control may be the feeding of heat-treated cereal grains. Apparently, the process of extruding feeds also results in killing of sporocysts. However, this is not possible in situations where horses are on pasture or are being fed hay. It is known that effective vaccines for protozoal diseases are difficult to produce. Now that this disease has been successfully reproduced in the laboratory, trials can be carried out to determine if vaccination would be efficacious. Hopefully this information will be forthcoming in the not too distant future.
EPM investigators at Ohio State have conducted considerable research on the disease since 1993, most recently completing the Seroprevalence and the Natural Infection studies, which are in the process of publication.
Our group's currently work focuses on the Risk Factor study, and we expect to pursue research on EPM test validation and a Multi-Center risk factor study.
The investigators are in the final stages off completing a retrospective case-control study intended to examine risk factors for disease. Two hundred and fifty EPM cases admitted to The OSU Veterinary Medical Center between 1992 and 1995 were enrolled in the study, as were 2 control series. One control series consisted of horses diagnosed with neurologic diseases other than EPM (neurologic control series, n=250), and the other control series were horses that were admitted for reasons other than neurologic disease (non-neurologic control series, n=250). Information regarding individual horse characteristics were obtained from hospital records and differences in rates among EPM cases and controls have been analyzed. Information regarding environmental exposures prior to admission is still being collected by telephone follow-up, as is information regarding survival and response to therapy among EPMcases.
Among horses with neurologic disease, those that are seropositive are 30 times more likely to have detectable antibody in CSF when compared to seronegative horses. Preliminary analysis of data from this retrospective study suggests that there is a significant difference between EPM cases and controls with regard to age, breed, and season at the time of admission.
Age was categorized into 10 discrete levels and examined as a risk factor for EPM utilizing the non-neurologic control horses as the comparison series. There was a significant linear trend of decreasing risk from youngest to older horses. When compared with horses less than 1 year old, the risk for EPM in 3 year olds was 22 times greater; the risk for EPM in 4 year olds was 18 times greater; and the risk among yearlings was 16 times greater. This is consistent with other infectious diseases in which the risk of disease is greatest among animals that have not acquired protective immunity through exposure.
We then examined the association between age and the occurrence of EPM using the neurologic horses as the control series. In this comparison there was a significant linear trend; this time risk increased from youngest to older horses. When compared to the horses <1 year of age, the risk for EPM in horses >14 years-of-age was 9 times greater; the risk among horses 10 to 13 years-of-age was 7 times higher; and the risk among yearlings was 5 times higher. Horses with EPM were significantly older than horses in the neurologic control group. This suggests that other common causes of neurologic disease are not manifest among older horses in the hospital population while EPM is seen in a broader range of ages.
We also examined the association between breed and the risk of EPM. When using the non-neurologic control series and the small breeds such as ponies, and miniature horses as the reference group, the risk for EPM was 7 times greater in Warmbloods, Thoroughbreds, and in Standardbreds. The risk for EPM among Quarter Horses was only 4 times higher than the reference group. There was not an association between breed and EPM when using the neurologic controls as the comparison series.
The association between season and the risk of EPM was examined categorizing January to March as Winter, April to June as Spring, July to September as Summer, and October to December as Fall. Using horses admitted during Winter as the reference group, horses admitted in the Fall were 4 times more likely to have EPM, and horses were twice as likely to have EPM if admitted in the Spring or Summer. This seasonal difference in admission rates was similar using both control series in comparisons. Assuming most cases have a short incubation period as has been indicated from experimental infection with S. falcatula, this suggests that there may be greater exposure to S. neurona sporocysts during warm weather.
Further information needed to finalize this study is currently being gathered by the investigators. However, preliminary results suggest that risk factors for EPM include age, breed, and season. In contrast to the seroprevalence where the rate increases with age, it appears that young horses have a much greater risk of EPM than do older horses, although this may be related to the number of horses in a particular age group presented to a veterinary hospital. The highest risk of presentation for EPM was seen among 3 year olds and may be partially related to athletic activity. Young horses represent the predominant proportion of those used in the racing and show horse industry.
Seasonal variations in the incidence of EPM has not been previously reported. The validity of this finding is supported by findings of our previous investigation that seroprevalence is affected by climactic conditions. If there is less exposure to the parasite in winter, this may explain the lower incidence of the disease at that time. Perhaps the organism numbers increase during the summer weather conditions which leads to higher exposure levels and greater risk for disease. Also, horses are often less stressed in the winter, which may explain part of this difference. The highest risk of disease was seen in the fall of the year, which may have been related to the stress of athletic performance at major competitions or year ending races.
Finally, it appears that there are risk factors for EPM not previously reported. It also is apparent there are likely several risk factors that have yet to be elucidated. The retrospective study will be completed by early 1997 and will provide information crucial to the proper conduct of a prospective study. Further work needs to be performed such as that proposed herein to improve our understanding the epidemiology of this devastating disease. This will hopefully lead to the development of control measures for prevention of EPM.
Little is known about the period of time between exposure to S.neurona and the manifestation of clinical signs in horses. The purpose of another study performed by our group was to follow the clinical course of horses naturally exposed to this parasite. All horses on a research facility were examined for evidence of neurologic disease and serum was analyzed using Western blot to detect antibody to S. neurona. At the initial screening, >80% of the 44 horses were seropositive, therefore only 6 horses could be used for this study.
Exposure to this farm environment prior to initiation of this study varied from 1 to 10 years for these 6 horses,. Horses were examined biweekly and samples of serum and CSF were obtained at this time. All horses developed an antibody response in the serum within 20 days except 1 horse which did not become seropositive until 4 weeks later. All horses were seropositive to S. neurona for greater than 50 weeks, and in 4 of 5 cases for 67 weeks. The presence of S. neurona antibody in serum for the long periods in these horses was likely due to either a protracted antibody response, a high initial antibody titer, persistence of the antigen in this aberrant host, or frequent re-exposures to the parasite. None of the horses developed clinical signs of EPM.
The prevalence of EPM has been estimated to be approximately 1% (D. Granstrom; Personal Communication). However, we know from previous investigations that there is a much higher exposure rate to S. neurona.[Saville, 1996 #142] Horses that are frequently being re-exposed to the parasite may develop a protective immunity which may explain the lack of clinical disease in this group of horses. The evidence for re-exposure includes the variability in exposure time, the high seroprevalence on this farm, the fact that all 6 horses became positive at the same time, and only 1 horse was seronegative after 67 weeks.
Another possibility is that horses in this study had not been exposed to the necessary stresses or risk factors required to produce clinical disease. This study suggests that the incubation period of EPM can be as long as a year or more, although there may be other factors required to trigger the disease process. The results of this study suggest further research into risk factors for EPM is necessary.
There had been limited data collected on the prevalence of S. neurona-specific antibodies in horses. The purpose of our first study was to determine the prevalence of serum antibodies to S. neurona in horses in Ohio.
Although data have been published on the prevalence of serum antibodies to Sarcocystis neurona-specific antigens in horses, some reports indicated that the seroprevalence was approximately 20%, based on horse farms in Ohio and central Kentucky. A seroprevalence rate of greater than 50% was reported for greater than 1,000 horses randomly selected from serum submitted for EIA testing in Ohio. This seroprevalence rate is considerably higher than that documented in previous studies, however, indications are that horses from a county in Pennsylvania revealed a seroprevalence rate of greater than 40%. Reports from the State of Oregon indicated seroprevalence rates between 20 and >60% from different regions of that state (Linda Blythe; Personal Communication).
Age was associated with seroprevalence of antibodies to S. neurona in horses in Ohio. The youngest seropositive horse in our study was 5 months of age. This is not surprising since several reports have indicated that foals as young as 2 months of age have had EPM. There was a linear relationship between age and seroprevalence indicating that as horses age, the risk for exposure increases. There was not an effect of gender on the seroprevalence of antibodies to S. neurona which is consistent with EPM.
Breed did not affect seroprevalence of antibodies to S. neurona, however, there was considerable variation between the breeds. The highest seroprevalence was in the Draft Breeds (>65%). There appeared to be an effect of location on seroprevalence of antibodies to S. neurona. Northeast Ohio had a seroprevalence rate of >45%, while the southwest area had a seroprevalence rate of >61%. While studies have not been performed to examine the effect of climate or soil conditions on S. neurona sporocysts, this has been examined with other Sarcocystis spp There appeared to be an effect of freezing days which correlated to different areas of the State of Ohio. Seroprevalence increases from the north group of counties to central to south. Although this would only affect sarcocysts in the intermediate host and scavenger transmission, this may explain our difference in seroprevalence from north to central to south Ohio.
In conclusion, the seroprevalence rate of antibodies to S. neurona in horses in Ohio was >50%. This was considerably higher than previously reported or anticipated. A high seroprevalence such as that found in our study suggests that the organism is ubiquitous in the environment and therefore, control of this disease is going to be very difficult. This further demonstrates the importance of examining cerebrospinal fluid for S. neurona-specific antibodies in attempting to diagnose EPM.
Livestock Movements and Disease Epidemiology in Chad Basin: Modeling Risks for Animals and Humans
A pilot study that examines livestock movements and disease epidemiology in the Far North Region of Cameroon. In the pilot study, we are examining the complex relationships between the different forms of livestock mobility and the transmission of zoonotic diseases in the context of the Far North Region. The pilot study is allowing us to collect baseline data on livestock mobility and prevalence of zoonotic diseases among humans and animals, test new methods, and build preliminary spatially-explicit models.
Foot-and-Mouth Disease Ecology and Livestock Movement in the Chad Basin
The goal of this project is to understand the ecology of infectious diseases in the context of pastoral mobility in the Lake Chad Basin. Specifically we want to know how livestock movements affect the ecology of foot-and-mouth disease (FMD) and the Foot-and-Mouth Disease Virus (FMDV).
Poopy Cows
The goal of this project is to construct an in silico dairy farm to test disease transmission among typical North American dairy cattle.
EPM Testing
Table of Contents
- Contact
- Summary
- Introduction
- Reasons for False Test Results
- Evaluating the Reliability of Diagnostic Tests
- The Effect of Disease Prevalence on The Reliability of Test Results
- Using Disease Prevalence Estimates to Aid in Interpreting Diagnostic Tests for EPM
- Conclusions
- Recommended Reading on the Clinical Epidemiology of Diagnostic Tests
- References
Contact
Antoinette E. Marsh, JD, MS, PhD
Department of Veterinary Preventive Medicine
The Ohio State University
A192 Sisson Hall
1920 Coffey Road
Columbus, Ohio 43210
Summary
We consider Western blot analysis of CSF a reliable method of diagnosing Equine Protozoal Myeloencephalitis in horses that have clinical neurologic disease. However, results of diagnostic tests must always be interpreted in light of the prevalence of disease in horses with similar clinical signs and exposures. False positive test results are common when the prevalence of disease is low while false negative results are common when prevalence is high. There is evidence to suggest that only a low percentage of clinically normal horses have detectable concentrations of antibody to S. neurona in their CSF. This low prevalence is expected to lead to a large proportion of false positive test results in this type of horse. Therefore, Western blot analysis of CSF obtained from clinically normal horses is not a reliable method of determining exposure status in individual clinically normal horses.
Introduction
Diagnostic tests are used widely in medicine to aid clinicians in effective diagnosis and treatment of disease. In recent years, advanced microbiologic techniques have given rise to an increasing number of tests which can be used to reliably aid in the diagnosis of disease. However, no diagnostic test is perfect and it is critical to understand test sensitivity, specificity, and predictive values in order to better understand when a test result may be true and alternatively when a result may be false. Nowhere in veterinary medicine is this more true than when practitioners use antemortem tests to aid in diagnosis of Equine Protozoal Myeloencephalitis (EPM).
Equine Protozoal Myeloencephalitis is a significant cause of neurologic disease of horses.1-5Sarcocystis neurona is a protozoan parasite which appears to have a predilection for infecting the central nervous system in horses and is thought to be the agent which causes Equine Protozoal Myeloencephalitis.1-5 Recent investigations suggest that the genome of S. neurona recovered from affected horses is nearly identical to that of Sarcocystis falcatula, a parasite whose definitive host is the opossum (Didelphis virginiana).6, 7 Currently, the most widely applied antemortem diagnostic test uses Western blot analysis to identify antibodies to S. neurona. Horses are commonly exposed to this parasite in geographic regions which the opossum inhabits. Surveys of horses from these regions have found that seroprevalence to S. neurona is often 50% or greater.8-10 Use of the Western blot assay to test for EPM relies on the assumption that antibodies to S. neurona are only produced intrathecally when the organism has infected tissues of the central nervous system. Comparison of results of Western blot analysis of CSF to results of rigorous postmortem examination of horses with neurologic disease suggests that the Western blot is approximately 89% sensitive and 89% specific for diagnosis of EPM (Dr. David Granstrom, personal communication, 1997).
The Western blot assay has been available on a commercial basis since 1992, and is used with confidence by many practitioners to aid in diagnosis of EPM. This is due in part to extensive validation and application of the Western blot. However, anecdotal reports of seemingly spurious results continue to cause controversy and frustrate clinicians. For example, it has become a common practice to collect CSF samples from yearling horses immediately after sales and submit them for testing using the Western blot assay. Some anecdotal reports suggest that as many as 16 out of a group of 20 clinically normal yearling horses may test positive for antibody to S. neurona in their CSF (Dr. David Granstrom; Personal Communication, 1996). It is very difficult to make appropriate recommendations regarding these results without understanding how the rate of false test results is affected by the sensitivity, specificity, and predictive values of the Western blot assay. Confusion about proper interpretation of this test is common as is illustrated by discussion among clinicians who subscribe to the Equine Clinicians Network (ECN), a popular E-mail based discussion group. The validity of the Western blot is a regular topic of discussion on this mailing list and a high level of frustration is evident among equine clinicians. These concerns could be partially alleviated if clinicians had a better understanding of the principles of clinical epidemiology related to diagnostic testing.
Reasons for False Test Results
Many veterinarians believe that false test results are most commonly caused by problems with the testing procedure. However, the reliability of diagnostic tests can be affected by several factors which can be broken down into four broad categories: problems with the biological marker that is measured by the test, differences among individual patients, problems with sample collection or storage, and problems with the testing procedure.
Problems with the Marker of Disease: Diagnostic tests are meant to identify some characteristic or marker of disease that is not found in healthy animals. An ideal marker of disease would be found in every affected animal early in the disease process as well as throughout its course, and would never be found in any unaffected animals. However, no marker of disease is perfect, and the usefulness in differentiating between healthy and diseased animals varies among different markers of disease. In addition, some tests assay for general markers of inflammation or disease, while others are designed to detect markers of very specific diseases. There are disadvantages to using both types of markers. Markers which identify generalized disease states may not be helpful when symptoms can be caused by many different conditions, particularly if the diseases require very different treatments. For example, CSF protein concentration, as well as CK and AST activities in CSF can be used as indicators of CNS inflammation and disease, but they cannot be used to specifically differentiate EPM from other diseases which cause similar clinical signs. Polymerase chain reaction (PCR) and monoclonal antibodies can be used to identify very specific markers of infection, but variability in the genomic and antigenic makeup of pathogens can potentially cause these diagnostic tests to yield results which do not reflect the true disease status of the patient.
Differences Among Individual Patients: Because of biologic variation, some healthy animals may express a marker which is usually only expressed by diseased animals, and some diseased animals may not express this marker when most others do. The degree that markers of disease are expressed may also vary among horses in different stages of disease (acute versus chronic). For example, clinicians have reported that horses in the very early stages of EPM may not have detectable concentrations of antibody in the CSF, but will be positive if retested a week or two later.2, 11, 12 The occurrence of other diseases can also affect the results of tests. For example, conditions affecting the integrity of the blood-brain barrier can allow serum antibody to leak into the CSF and thus affect results of diagnostic tests for EPM.
Problems with Sample Collection or Storage: Veterinarians frequently obtain cerebrospinal fluid from the lumbosacral or atlanto-occipital spaces for the purposes of diagnostic testing. However, it is easy to inadvertently contaminate samples with blood during the sampling procedure which can cause CSF samples to have falsely elevated S. neurona antibody concentrations. This is particularly problematic because of the high seroprevalence to S. neurona.8-10 This is why the CSF indices (albumin quotient and IgG index) are used to aid in determining if antibody in CSF was produced intrathecally.13-15 Inappropriate handling during sampling or storage can damage samples and increase the likelihood that false test results will be obtained. For example, immunoglobulin and other proteins can be denatured by exposure to extreme heat or UV radiation.
Problems with the Testing Procedure: Inherently, some diagnostic tests have more variability than others and it may require considerable skill and finesse to produce consistent results. The variability in skill and diligence used when performing diagnostic tests can easily affect results, as is true of any technical task. It is worthwhile to mention that variation in diagnostic test results can be manifest as inaccuracy as well as imprecision. Differences between these two types of variability are often illustrated by correlating multiple test results with the pattern that a shotgun might make on a target. Accuracy describes how well the pattern is aligned with the center of the target. Precision describes how small the pattern is. Known standard samples should be tested with every batch in order to ensure a high degree of accuracy between batches. Procedures should be strictly standardized whenever possible to increase the accuracy and precision between batches.
Evaluating the Reliability of Diagnostic Tests
In order to estimate the usefulness or reliability of a new diagnostic test, results are compared to the true disease status of a population of animals. Parallel analysis of samples using two different assays allows calculation of the new test's sensitivity and specificity. Unfortunately, it is not possible to be 100% confident of the disease status of any animal, and so results of the new test are usually compared to those of an accepted method of diagnosis, a "gold standard." It is important to emphasize that results of the gold standard only provide a different estimate of the true disease status, and results will in fact not be correct in some cases. However, for purposes of comparison, this standard is used to provide the best or most practical estimate of true disease status that is available.
In epidemiologic terms, sensitivity and specificity describe the rate of false positive and false negative results that will be obtained from a diagnostic test. SENSITIVITY is the proportion of truly diseased animals that test positive, while SPECIFICITY is the proportion of non-diseased animals that test negative (Figure 1). In other words, a test that has a high probability of correctly identifying diseased animals has a high sensitivity, and a test that is highly likely to confirm that healthy animals are not diseased has a high specificity. Using the estimate cited previously for the sensitivity and specificity of Western blot analysis of CSF when used to diagnosis EPM, 89% of horses with post-mortem findings suggestive of EPM would be expected to have detectable concentrations of antibodies in their CSF, and 89% of horses with other neurologic diseases would be expected to test negative using Western blot analysis of CSF. Unfortunately, tests that are extremely sensitive will often have a lower specificity, and tests that are extremely specific often have a lower sensitivity. Tests that are both highly sensitive and highly specific are often expensive or technically difficult to perform. It should be noted that microbiologists sometimes use the terms sensitivity and specificity in a different way: microbiologic sensitivity can be described as the lower limit of detection of a diagnostic test (i.e., the lowest antibody concentration can be detected), and specificity is used to describe whether a diagnostic test will detect exposure to several different microorganisms.
Figure 1.
Test Results | True Status (+)* | True Status (-)* | Totals |
---|---|---|---|
(+) | True Positive (a) |
False Positive (b) |
Test Positive Animals (a+b) |
(-) | False Negative (c) |
True Negative (d) |
(c+d) Test Negative Animals |
Disease Positive (a+c) |
Disease Negative (b+d) |
All Animals (a+b+c+d) |
*True Disease Status ("Gold Standard" Test Results).
Test Sensitivity
Proportion of True Positive results among Disease Positive animals = a / (a+c)
Test Specificity
Proportion of True Negative results among Disease Negative animals = d / (b+d)
Positive Predictive Value
Proportion of Disease Positive animals among with those with Positive Test results = a / (a+b)
Negative Predictive Value
Proportion of Disease Negative animals among those with Negative Test results = d / (c+d)
True Prevalence
Proportion of all animals that are truly diseased = (a+c) / (a+b+c+d)
Apparent Prevalence
Proportion of all animals that are Test Positive = (a+b) / (a+b+c+d)
The Effect of Disease Prevalence on The Reliability of Test Results
Most practitioners understand that a test with high sensitivity and specificity is more reliable than a test with low sensitivity and specificity, but few are able to extend this understanding to aid in interpretation of results of diagnostic tests for individual patients. Sensitivity and specificity describe the proportion of truly diseased animals that will test positive, and the proportion of truly healthy animals that will test negative. However, this is not exactly the information that a practitioner needs when interpreting test results for a patient. The clinician actually needs to know what proportion of animals that test positive are truly diseased (POSITIVE PREDICTIVE VALUE), and what proportion of animals that test negative are not diseased (NEGATIVE PREDICTIVE VALUE) (Figure 1). The difference between sensitivity and positive predictive value (PPV), as well as the difference between specificity and negative predictive value (NPV) may seem subtle, but are extremely important. While sensitivity and specificity are fixed characteristics of a test that will remain the same when different populations of animals are tested, positive and negative predictive values are greatly affected by the true prevalence of disease in the population. Figure 2 shows how the positive predictive value for a test with 89% sensitivity and 89% specificity changes as the prevalence of disease changes. The positive predictive value is very low when disease prevalence is low and is maximized when prevalence is high. This means that when using the Western blot to diagnose EPM, the rate of false positive and false negative test results will change depending upon the symptoms of disease that are shown by patients.
Figure 2.
Changes in positive predictive value relative to changes in the prevalence of disease for a test that is 89% sensitive and 89% specific.
It may seem odd that the ability of a test to predict the presence or absence of disease (predictive value) changes even though the sensitivity and specificity of a test are fixed characteristics. The reason that this happens is illustrated using three hypothetical populations with disease prevalences of 95%, 50%, and 1% in Figure 3, Figure 4, and Figure 5, respectively. The sensitivity and specificity is fixed in these examples (89% and 89%), but the positive and negative predictive values are markedly different (respectively, PPV=99%, 89%, 8%; NPV=31%, 89%, 100%). Remember that the proportion of true positive and false positive test results determines the positive predictive value, and animals with false positive test results do not actually have disease. In situations where the disease prevalence is high (and most of the animals are truly diseased), only a minority of animals are disease negative so that the number of true positive test results far outweighs the number of false positive results and the positive predictive value will be high (Figure 3). If the true disease prevalence is low (and most animals are not diseased), the actual number of false positive results far outweighs the number of true positive results even though the proportion of each is determined by fixed sensitivity and specificity, and the positive predictive value will be low (Figure 5). Notice when the true prevalence of disease is 1% (Figure 5), the apparent prevalence of disease (i.e. the proportion of all animals that test positive) is very different from the true prevalence of disease (12% versus 1%). Negative predictive value is similarly affected by disease prevalence, except that it is maximized when disease prevalence is low (Figure 3 and Figure 5).
Figure 3.
Test Results | True Status (+)* | True Status (-)* | Totals |
---|---|---|---|
(+) | 846 | 4 | 850 |
(-) | 104 | 46 | 150 |
950 | 50 | 1000 |
*True Disease Status ("Gold Standard" Test Results).
- Sensitivity: 89%
- Specificity: 89%
- True Prevalence: 95%
- Apparent Prevalence: 85%
- Positive Predictive Value: 99%
- Negative Predictive Value: 31%
Figure 4.
Test Results | True Status (+)* | True Status (-)* | Totals |
---|---|---|---|
(+) | 445 | 55 | 500 |
(-) | 55 | 445 | 500 |
500 | 500 | Total 1000 |
*True Disease Status ("Gold Standard" Test Results)
- Sensitivity:89%
- Specificity: 89%
- True Prevalence: 50%
- Apparent Prevalence: 50%
- Positive Predictive Value: 89%
- Negative Predictive Value: 89%
Figure 5.
Test Results | True Status (+)* | True Status (-)* | Totals |
---|---|---|---|
(+) | 9 | 109 | 118 |
(-) | 1 | 881 | 882 |
10 | 990 | 1000 |
*True Disease Status ("Gold Standard" Test Results)
- Sensitivity: 89%
- Specificity: 89%
- True Prevalence: 1%
- Apparent Prevalence: 12%
- Positive Predictive Value: 8%
- Negative Predictive Value: 100%
Using Disease Prevalence Estimates to Aid in Interpreting Diagnostic Tests for EPM
It may seem somewhat circular that prevalence must be accounted for in order to correctly interpret diagnostic test results: disease status must be known in order to estimate disease prevalence and yet, if the true disease status of the animals were known there would be no need to run the tests. However, it is not necessary to have exact knowledge of disease prevalence. Rough estimates of disease prevalence in similar animals can be used in order to make practical generalizations about the predictive value of diagnostic test results.
For example, it is possible to categorize horses into different risk groups for EPM (Figure 6). Approximately 50% of horses admitted to The OSU Veterinary Medical Center with neurologic problems are found to have detectable concentrations of antibodies to S. neurona in their CSF and are therefore diagnosed with EPM. Among horses in this population with detectable neurologic problems, the prevalence of EPM among horses that are seropositive is 67% (i.e., seropositive horses with neurologic disease are much more likely to have antibodies to S. neurona in their CSF than are seronegative animals with neurologic disease). Using information from post-mortem evaluation of horses with neurologic disease and available estimates of horse numbers, the overall population prevalence of clinical EPM has been estimated to be approximately 0.5-1% (Dr. David Granstrom, personal communication, 1997). It has been suggested that, in general, horses are unlikely to have antibody to S. neurona in their CSF without showing clinical signs.11 If this is true, then overall less than 1% of clinically normal horses would be expected to have detectable concentrations of S. neurona in their CSF.
When interpreting results of the Western blot analysis of CSF obtained in clinical situations, it is therefore possible to classify a patient using these three distinct categories and make generalized estimates of the predictive value of this diagnostic test for that individual animal (Figure 6). In other words, the credibility of information obtained from diagnostic tests can be weighted if the prevalence of disease in similar animals can be estimated.
If the horse is clinically normal the predictive value of a positive result from Western blot analysis of CSF is probably very low; if assumptions from this example are correct, the positive predictive value in clinically normal horses is 8% or lower (Figure 6). This means that only 8% of positive test results would be expected to correctly predict the presence of disease! However, if the clinician uses clinical skills to screen which horses will be tested using the Western blot, it is possible to use this test in situations that it is more likely to yield useful information. When the test is applied in horses presented to The Ohio State University Veterinary Medical Center with clinical neurologic disease, the prevalence of disease is expected to be approximately 50% and the predictive value of a positive test is approximately 89% (Figure 6). If the horse has detectable neurologic disease and is also seropositive the positive predictive value is even higher, approximately 94% (Figure 6).
Figure 6.
Type of Horse | Estimated True Prevalence of Horses with Antibodies to S. Neurona in CSF* | Apparent Prevalence (Proportion of horses with positive test results) | Positive Predictive Value |
---|---|---|---|
No Neurologic Abnormalities | 1% | 12% | 8% |
Neurologic Abnormalities Present | 50% | 50% | 89% |
Neurologic Abnormalities and Seropositive | 67% | 63% | 94% |
* Based upon the prevalence in horses presented to The Ohio State University Veterinary Medical Center. These values will be different in other populations depending upon the likelihood of exposure to S. neurona and other risk factors.
Conclusions
Further work is needed to corroborate the high sensitivity and specificity that has been reported for the Western blot assay when used to diagnose EPM. However, this test has been used extensively in the past five years for this purpose and available information suggests that it is highly sensitive and highly specific. It will not, however, be useful when used in horses where the disease prevalence is very low (Figure 6). Considering available information about the prevalence of disease, it is not recommended that this test be used in clinically normal horses to determine if individual animals have been exposed to S. neurona. This diagnostic test appears to be much more reliable when used in horses that have clinical signs which could be attributed to EPM (Figure 6).
Recommended Reading on the Clinical Epidemiology of Diagnostic Tests
Sackett DL, RB Haynes, Tugwell. Clinical Epidemiology: A Basic Science for Clinical Medicine, 2nd ed. 1991. Little, Brown, and Company, Boston/Toronto. 441 pp.
References
- Granstrom DE, Dubey JP, Davis SW, et al. Equine protozoal myeloencephalitis: antigen analysis of cultured Sarcocystis neurona merozoites. J Vet Diagn Invest, 1993;5:88-90.
- Granstrom DE, Dubey JP, Giles RC, et al. Equine protozoal myeloencephalitis: Biology and epidemiology. In: Nakajima H, Plowright W, eds. Proc VII Intern Conf Equine Infect Dis, Tokyo, Japan: R & W Publications Ltd., Newmarket, UK; 1994:109-111.
- Granstrom DE, Saville WJ. Equine Protozoal Myeloencephalitis. In: Reed SM, Bailey WM, eds. Equine Internal Medicine. Philadelphia, Pa.: WB Saunders Company; 1995:In Press.
- Reed SM, Granstrom DE. Equine protozoal encephalomyelitis. Proc Am Coll Vet Intern Med Forum. Washington, DC; 1993:591-592.
- Reed SM, Granstrom D, Rivas LJ, Saville WA, Moore BR, Mitten LA. Results of cerebrospinal fluid analysis in 119 horses testing positive to the western blot test on both serum and CSF to equine protozoal encephalomyelitis. Proc Am Assoc Equine Pract. Vancouver, BC; 1994:199.
- Fenger CK, Granstrom DE, Langemeier JL, et al. Identification of Opossums (Didelphis virginiana) as the putative definitive host of Sarcocystis neurona. J. Parasitol 1995;81:916-919.
- Dame JB, MacKay RJ, Yowell CA, Cutler TJ, Marsh A, Greiner EC. Sarcocystis falcatula from passerine and psittacine birds: Synonymy with Sarcocystis neurona, agent of Equine Protozoal Myeloencephalitis. J. Parasitol 1995;81:930-935.
- Saville WJ, Reed SM, Granstrom DE, et al. Prevalence of serum antibodies to Sarcocystis neurona in horses residing in Ohio. J Am Vet Med Assoc 1997;210:519-524.
- Blythe LL, Granstrom DE, Hansen DE, Walker LL, Bartlett J, Stamper S. Seroprevalence of antibodies to Sarcocystis neurona in horses residing in Oregon. J Am Vet Med Assoc 1997;210:525-527.
- Bentz BG, Granstrom D, Stamper S. Seroprevalence of antibodies to Sarcocystis neurona in horses residing in a county of southeastern Pennsylvania. J Am Vet Med Assoc 1996;210:517-518.
- Reed SM, Saville WJA. Equine Protozoal Encephalomyelitis. In: Zinninger SE, ed. Proc Am Assoc Equine Pract, Denver, CO; 1996:75-79.
- Miller MM, Bernard WV. Usefulness of cerebrospinal fluid indices and the polymerase chain reaction for Sarcocystis neurona in diagnosing equine protozoal myeloencephalitis. In: Zinninger SE, ed. Proc Am Assoc Equine Pract, Denver, CO; 1996:82-84.
- Andrews FM, Maddux JM, Faulk D. Total protein, albumin quotient, IgG and IgG index determinations for horse cerebrospinal fluid. P Vet Neuro 1991;1:197-204.
- Andrews FM, Provenza M. Differentiating neurologic diseases in the horse using albumin quotient and IgG index determination. In: DeNovo R, ed. Proc Am Coll Vet Intern Med Forum, 13 ed. Lake Buena Vista, Fl; 1995:600-603.
- Andrews FM, Granstrom DE, Provenza M. Differentiation of neurologic diseases in the horse by the use of albumin quotient and IgG index determinations. Proc Am Assoc Equine Pract, Lexington, KY; 1995:215-217.